Climate research and development
Rossby Centre is involved in national and international projects on climate modelling and research, and on the application of climate information for decision-making for adaptation as well as for measures that limit climate effects.
The research at Rossby Centre aims to increase knowledge about the future climate with a focus on societal benefits. It includes climatological, meteorological, oceanographic and hydrological aspects. The work includes a chain of activities with model development, climate simulations and evaluation of data for climate studies and climate services at SMHI, in Sweden and in Europe, climate information, as well as application of model data for impact and adaptation studies. Rossby Centre participates in national and international projects in climate modelling, climate research and the application of climate information.
Facts and knowledge from our research are used in decisions on measures to limit climate-change emissions to reduce negative climate effects and consequences for society and the natural environment, and to plan and adapt society to a new, changed climate in due time.
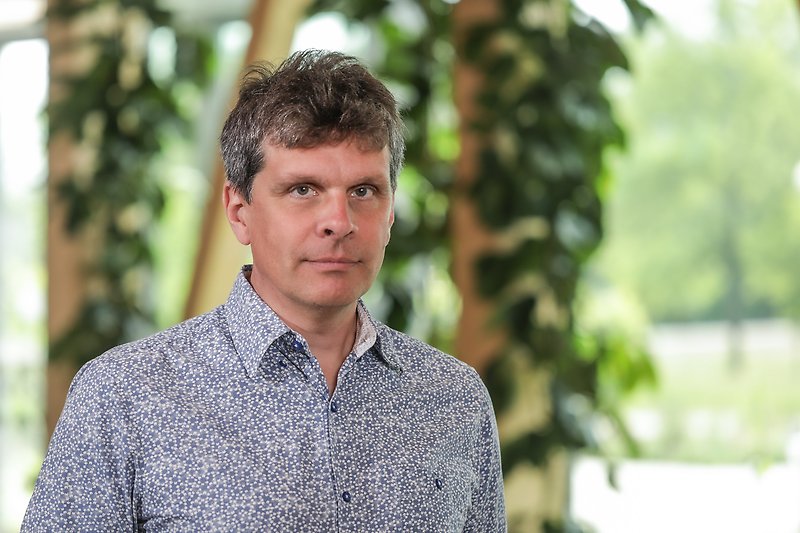
Find on this page
Within the Rossby Centre there are five strategically important research areas that cover signicifant aspects of climate research:
SEACLIM
SEACLIM (European SEAs CLIMate impact prediction through regional models) aim to forecast the future of our regional seas, with decadal predictions and long-term climate projections.AI4PEX
AI4PEX, Artificial Intelligence for enhanced representation of processes and extremes in Earth System Models, is a research project that will deliver enhanced knowledge on the Earth system by integrating Earth observations, artificial intelligence, and machine learning into Earth system modelling and analysis.TipESM
TipESM brings together scientists from a range of disciplines to deliver a step change in our understanding of climate tipping points in the Earth system, including their impact on ecosystems and society, combined with a set of early warning indicators and safe future emission pathways that minimise the risk of exceedi...OptimESM — Optimal high resolution Earth system models for exploring future climate change
New and better knowledge is needed to support sustainable decision-making on ongoing climate change and avoiding tipping points. OptimESM (and its new Earth system models (ESMs) will provide decision-makers with just that.FutureGS
How fast will the AMOC and thus the Gulf Stream decline in the future, how large is the risk for a rapid reduction and what would be the impact for the European climate? In the project FutureGS these questions will be answered.
Rossby Centre develops three-dimensional numerical models of the climate system. The models are based on mathematical descriptions of the physical interaction between processes in the climate system, and describes the interplay between atmosphere, sea, land and ice. The climate models are verified against observations of the climate up to today. The researchers also calculate how robust and reliable the results are with different assumptions about future greenhouse gas emissions, in order to increase the usefulness of the results.
Climate scenarios are produced with both global and regional climate models and are differently detailed at global, regional and local level. The climate scenarios can be used as a basis for society beneficial decisions and for further research.
The current regional climate model at Rossby Centre is HARMONIE-Climate, HCLIM, which is used for detailed climate scenarios. These include extreme events much more realistic than was possible in previous models. HCLIM will be expanded with coupled versions with ocean models and vegetation processes. The regional atmospheric model RCA is about to be phased out.
Rossby Centre has developed a global climate model EC-Earth3, which is used for international projects on global warming. Data and results from EC-Earth3 are included in the research that forms the basis for the conclusions presented in the latest report from the UN Climate Panel IPCC AR6. A new global climate system model, EC-EARTH4, is being developed in collaboration with a consortium of European weather services and universities and the European Centre for Medium Range Weather Forecasts.
Rossby Centre thus delivers climate knowledge, data and information as a scientific basis for decisions. This is done in collaboration with other groups within SMHI and in national and international projects.
Contact us at the Rossby Centre
The unit is led by Helena Martins, together with Ralf Döscher and Torben Koenigk.
Rossby Centre arranges the Swedish Climate Symposium
We publish our research in international peer-reviewed journals and in SMHI reports. The two most recent publications are shown below.
Projected changes in near-surface wind speed in the Arctic by a regional climate model
Mirseid Akperov, Wenxin Zhang, Torben Koenigk, Alexey Eliseev, Vladimir A. Semenov, Igor I. Mokhov
Navigating towards strengthened climate service processes
Lotten Wirehn, Gustav Strandberg
SMHI convened conference debate for young researchers
Last week a group of SMHI colleagues attended EGU, the European Geosciences Union annual conference in Vienna. Helena Martins, head of climate research at SMHI, convened a panel debate focusing on how young researchers can navigate the policy-research divide and have an impact on society in their early career.Swedish Climate Symposium 2024 – registration open
On May 15-17 it is time for a new edition of the Swedish Climate Symposium in Norrköping. The focus is climate research for society and we welcome both researchers and practitioners to join, for three days centered around understanding the science, investigating implications and exploring responses. Register by 15 Apri...Now you can submit your contribution to the Swedish Climate Symposium
In May 2022 SMHI co-organised the first ever The Swedish Climate Symposium. The symposium offered interesting sessions, opportunities to meet and a visit from HRH Crown Princess Victoria. In May 2024 it is time for a new symposium and now you can submit your abstract.